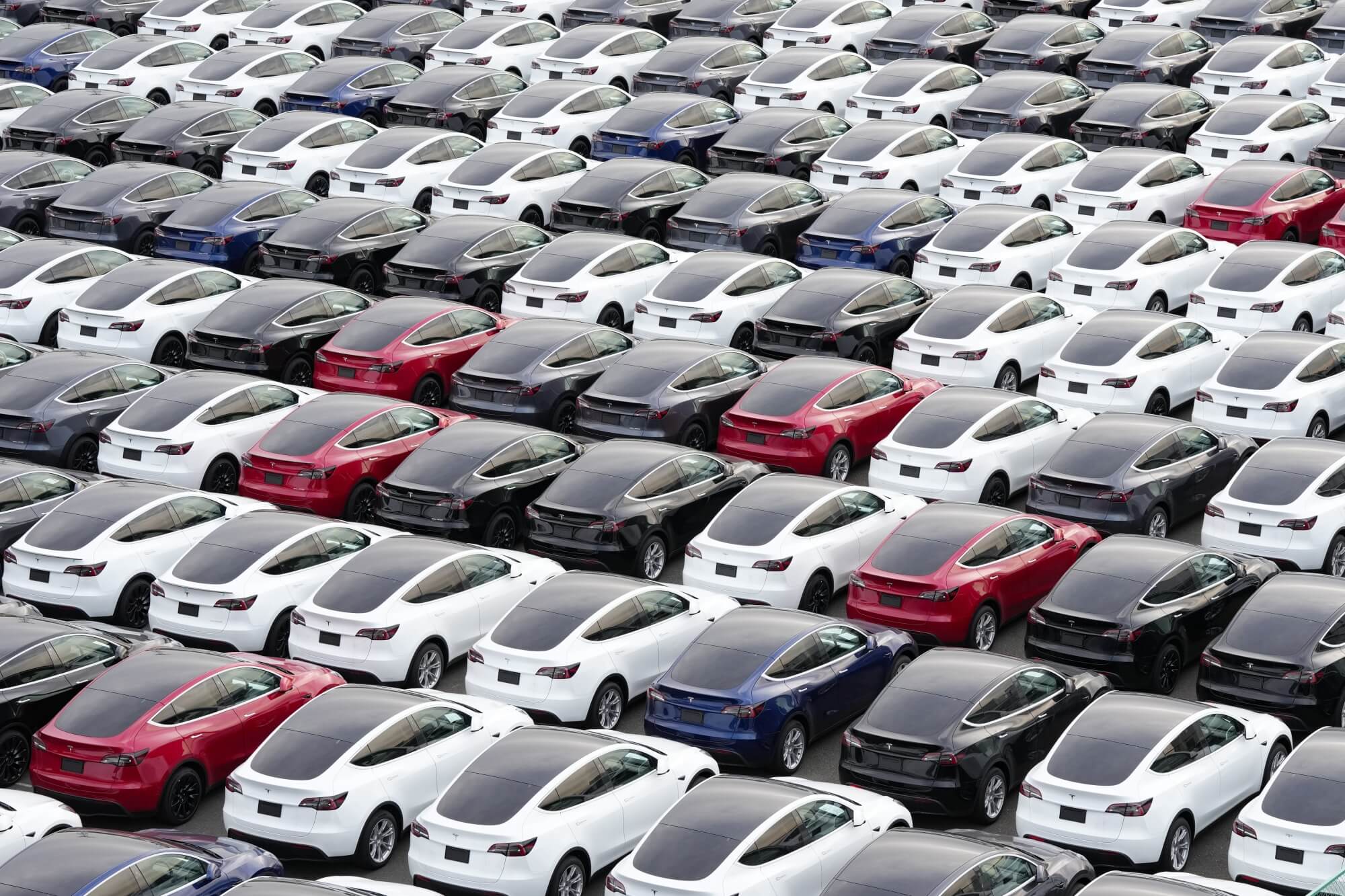
Markets
Market
Overview
Size and Growth
A $18 BILLION MARKET GROWING AT 30%
The SiC device market is expected to reach $18bn by 2029, driven by the surging adoption of EVs and continued strong growth in other sectors such as wind and solar energy and battery storage.
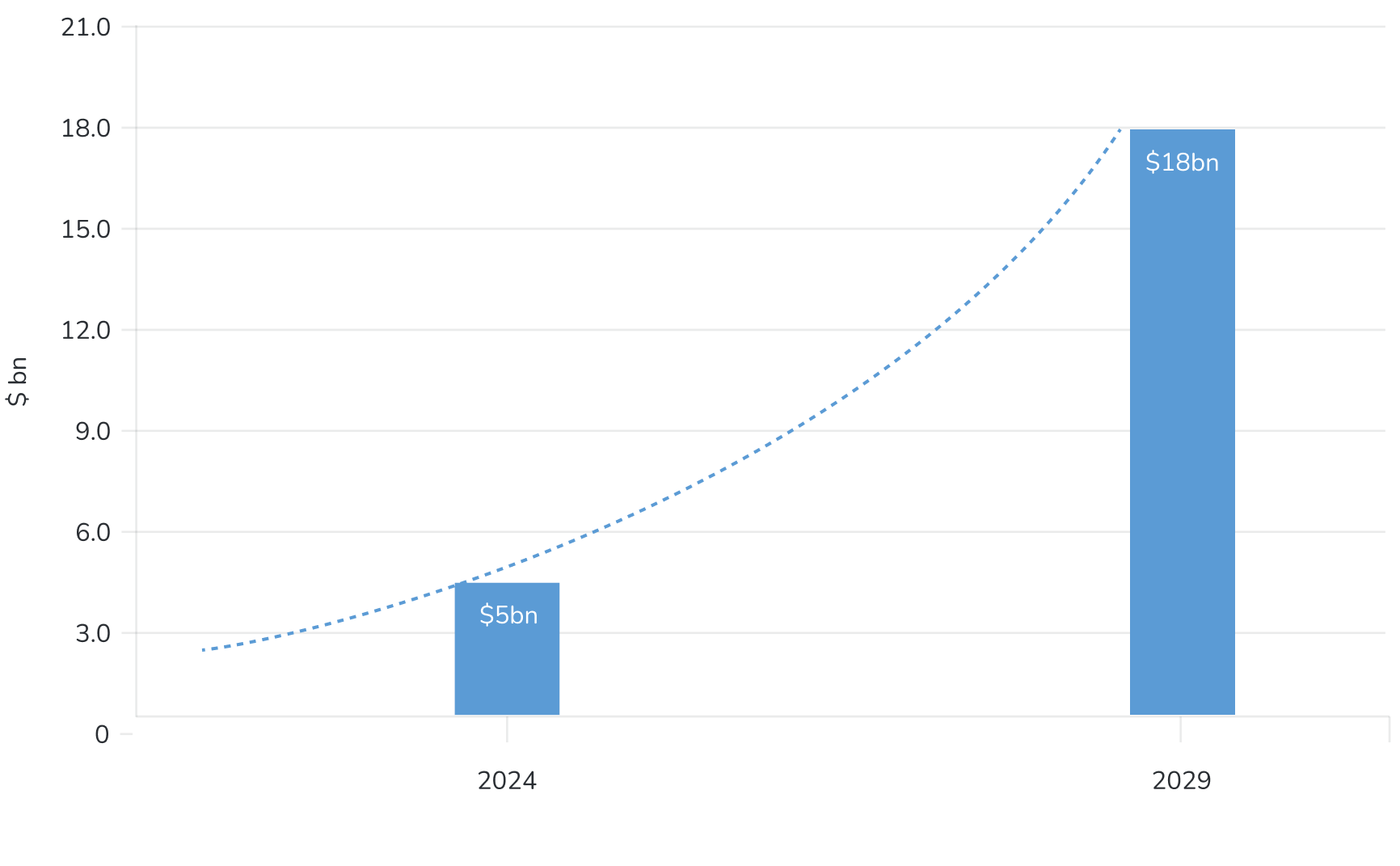
SiC Device Market Size, 2023
Advantages of SiC
Compared to silicon, SiC has four fundamental properties that make it a superior semiconductor material on which to make both power chips and RF chips:
- Wider band gap
- Lower on-state resistivity
- Higher thermal conductivity
- Higher operating frequency
In simple terms, wide band gap means that a chip can tolerate higher temperatures before it loses its semiconducting capabilities and becomes just a conductor, and is therefore no longer able to control or convert power. Lower on-state resistivity means higher power can pass through a chip with less of it being converted to and lost as heat. Thermal conductivity on the other hand is the ability of a chip to dissipate heat.
Silicon has a narrow band gap and low thermal conductivity. When high power is passed through a silicon chip, it begins to heat up because of its higher resistance, and it cannot dissipate this heat. Its temperature begins to rise, and it only takes a moderate temperature rise for the silicon chip to then lose its semiconducting capabilities. In order for silicon chips to handle the high power levels typically associated with systems such as electric vehicles, large, heavy and costly cooling systems have to be included. Regardless, a significant amount of power is converted to and lost as heat.
By contrast, an SiC chip can handle high power throughput without heating up to the higher temperatures at which it would lose its semiconducting capabilities. More importantly, it is able to handle the high throughput with approximately 50% lower energy-to-heat losses than a silicon chip. Over the lifetime of an EV, this translates into huge savings in charging energy (and bills) required to achieve the same driving distances.
When it comes to operating frequency, this simply means that electrons move faster through SiC than Si and an SiC chip can move between states, such as on or off, more rapidly than silicon. One of the core objectives of modern communications technologies such as 5G is to increase the rate of data transfer by utilizing higher frequency bandwidths of the Radio Frequency (RF) spectrum. Here again, SiC chips excel in both generating and decoding high frequency signals.
Applications
The fundamental properties of SiC make it particularly advantageous for use in power and RF chips.
Power
There are two basic types of power chips known as diodes and transistors and they perform three main functions:
- Diodes directly convert alternating current (AC) to direct current (DC) and work together with transistors to convert DC to AC
- Transistors turn power on and off
- Transistors also increase or decrease the voltage of power
SiC Schottky Diodes and MOSFET Transistors perform these functions with significantly lower energy losses than their silicon counterparts, and in significantly smaller and lighter form factors. This makes them the preferred choice for applications such as EV chargers where the convert AC from the grid to DC for the battery, and inverters, where they convert DC from the batter to AC for the electric traction motor.
Schottky diode
MOSFET
RF
Amplifiers, generators and frequency converters incorporating SiC semiconductors facilitate high data transmission rates in 5G, lightweight radiation-proof electronics in satellites, and more powerful and precise radar systems.
Industries
Key industries that are undergoing rapid innovation due to the superior properties of SiC semiconductors include Transportation, Energy, and Communications.